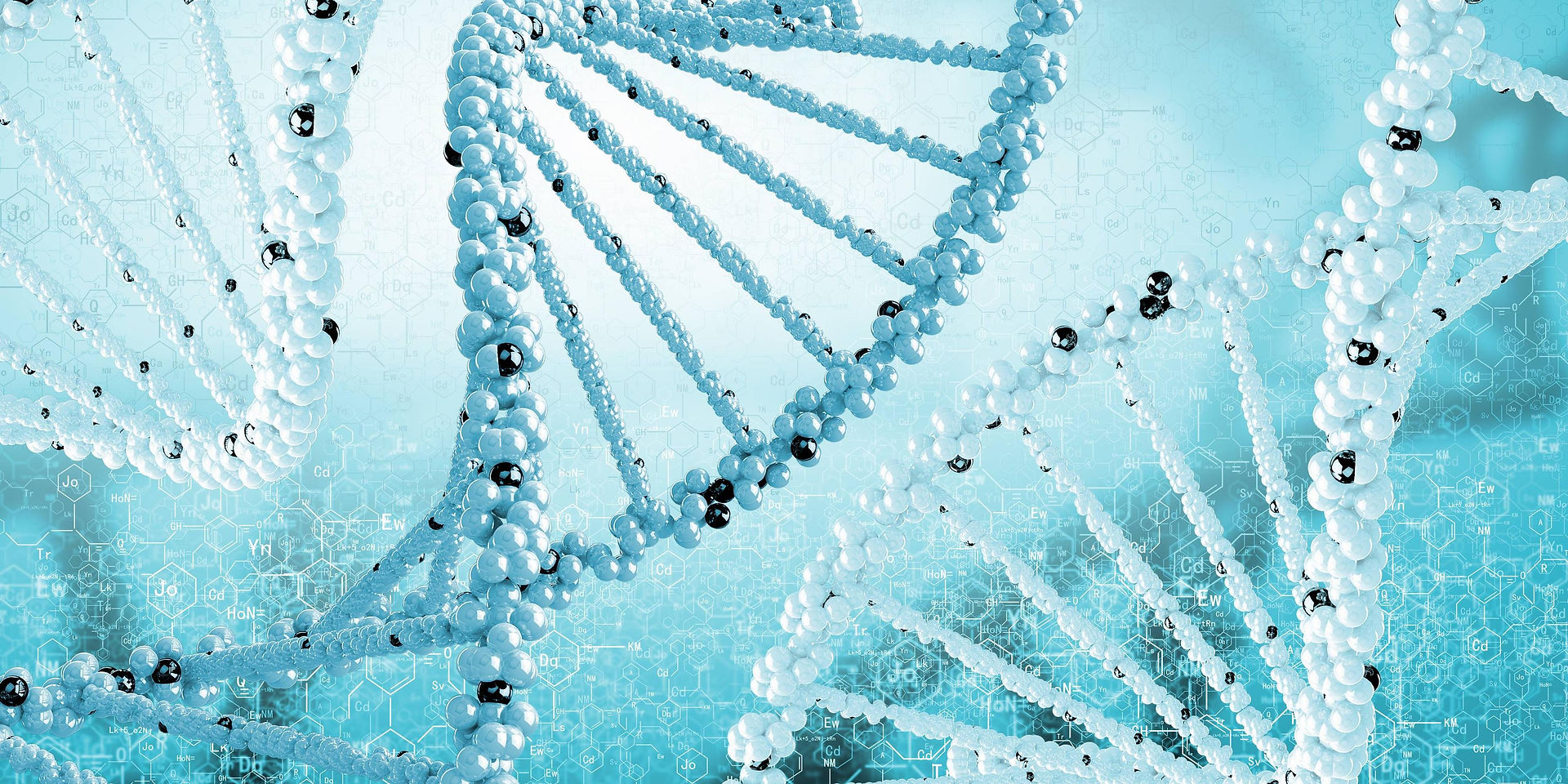
DNA: The Root of All
It comes with no surprise that to understand DNA sequencing, one has to understand the DNA first. The video at the left summarizes some of the basics to get us started.
The Sequence of Sequencers: The History of DNA Sequencing
First Generation Sequencing
Around 1953, Watson and Crick developed the first ever model of the DNA, giving the world inherent information of the most valuable asset to their unique identities. They took crystallographic data from Rosalind Franklin and Maurice Wilkins which alongside giving them a framework for the DNA, gave them information about the replication process and how proteins are coded. However, due to DNA’s infinite length and the small number of nucleotide pairs, it became hard to configure the sequences properly.
Since DNA was a rather large piece to use as a mechanism for finding the order of the bases, scientists opted for ribosomal RNA or transfer RNA in microbials or used RNA from bacteriophages which were all much shorter than a typical eukaryotic cell and did not have the complementary strand like DNA. However, things began to slow down when instead of figuring out the actual order of the base pairs, scientists were only able to figure out the composition which marked the entry of Robert Holley in 1965. Holley and his colleagues used alanine tRNA from Saccharomyces cerevisiae to develop a nucleic acid sequence. Alongside Holley, Fred Sanger, a British biochemist used DNA fractionation to add to the existing ribosomal and transfer RNA. Fractionation occurs through the use of a pulsed field electrophoresis with alternating electric fields to separate DNA fragments according to characteristics such as their mass. Around this time, scientists broadened the scope of their research and began working with purified bacteriophages which was made possible with the emerging research in the field of genomics. Since these organisms have five hanging cohesive ends, Ray Wu and Dale Kaiser used polymerase to create radioactive nucleotides at the end in order to deduce the sequence of DNA. Despite these attempts, the bases the scientists found turned out to be shorter than usual thus calling for more alterations.
​
In order to reach better results, new developments had to be made using DNA fractionation. Since this process involved both gel electrophoresis and chromatography, a two way technique using the protocols of Alan Coulson and Sanger’s plus and minus systems, and Allan Maxam and Walter Gilbert’s cleavage techniques were adopted. A primer is used to synthesize DNA with radiolabelled nucleotides which is ended with a plus reaction, or polymerization to create extensions that end with a single type of nucleotide. The minus test is where the other three nucleotides come into play and are sequenced until there is a gap in the sequence where the fourth nucleotide from the plus reaction is absent. Afterwards, gel electrophoresis is used to analyze where each of the nucleotides are at. Using this method, Sanger and his colleagues were able to find the genome of a bacteriophage. Despite their significant similarities, the Gilbert and Maxam approach differed in how the DNA fragments were cleaved. Instead of using polymerization, a chemical substance was used to break the nucleotides up into smaller pieces. This method was more prominent than Sanger’s method and is known to be the first true application of DNA sequencing.
In 1977, Sanger made another advancement in the field of DNA technology which drastically altered the manner in which sequencing was done prior to the discovery. Called the dideoxy technique, this method took advantage of the absence of a 3’ hydroxyl group which are needed for the extension of DNA chains thus connecting to 5’ phosphate groups. Using radiolabelled nucleotides at unequal concentrations with the DNA concentration, this slows down the process of DNA synthesis. This process is performed using DNA polymerase where DNA synthesis is performed. While doing so, modified pieces of nucleotides (dideoxynucleotides) of bases A, C, G, and T are added and since they lack a hydroxyl group, it stops the synthesis process midway. Since there are four bases, each reaction which involves the production of a DNA strand have to be given the four nucleotides separately. This process results in several different lengths of DNA fragments, each unique to its own. The next step is to denature the DNA fragment and to run it through gel electrophoresis. The simplicity and ease with which one could perform this process made it the universal method of DNA sequencing which came to be know as chain termination or simply, Sanger Sequencing.
The next few years followed up with improved techniques in this field, which was being able to incorporate more than one base in a single reaction as well as producing new machines which could identify sequences of complex species around the world. More methods that are readily used today began to be developed and resulted in what we know today as the polymerase chain reaction (PCR) and recombinant DNA technologies.
​
​
The picture at the right shows the process of a PCR technique: Polymerase Chain Reaction.
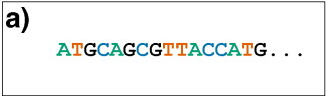
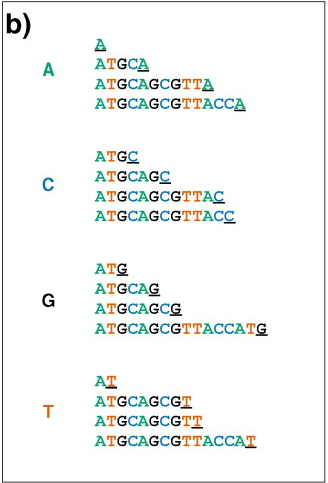
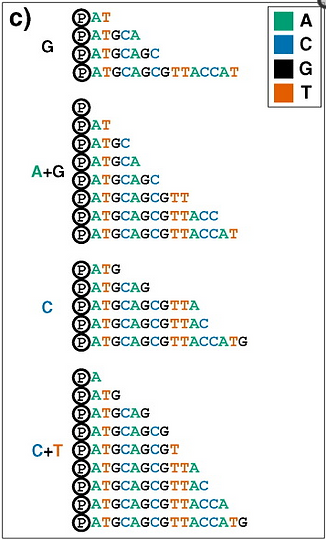
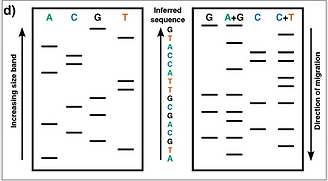
The pictures above show various methods of DNA sequencing. The first method (A) is the Sangher method, the second one (B) is chain termination method while the third one (c) is the Maxam-Gilbert method.
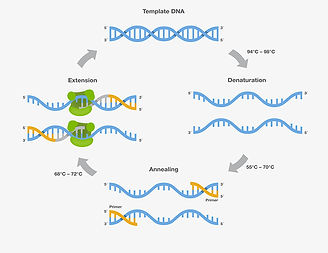
Second Generation DNA Sequencing
As time went on, researchers discovered new possibilities. Instead of relying on radiolabelling methods or using the four dideoxynucleotides, researchers looked into the luminescent method (producing light without heat). This method used a two tier method of conversion using enzymes. In the first round, ATP sulfurylase converts pyrophosphate into ATP which is then used as a substrate for luciferase which is an enzyme that produces light through bioluminescence. The amount of pyrophosphate produced would become the indicator of the sequence of DNA. These methods were extremely helpful because they did not require an artificial base and there wasn’t a need for any waiting around. Another technique involved using paramagnetic beads to connect it to the DNA strands which greatly reduced the steps involved with washing the DNA in order to remove unwanted bases. However, certain tests reaffirmed that this technique wasn’t so efficient after all since it bore more than one base at a time.
​
​
​
​
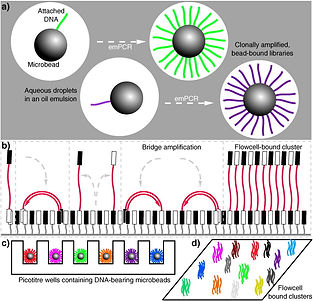
With the increasing number of DNA machines and novel ways of sequencing DNA, the number of DNA particles that could be used at a single time began to increase. In a particular example where the machine 454 is used, DNA is linked to beads using adapter sequences. Afterwards, these DNA pieces are run through a PCR process called water-in-oil emulsion. Each bead which was already present on the DNA strand get covered in a clonal DNA population so that each individual DNA molecule could take up place in the entire bead of emulsion and be amplified. Using a picoliter reaction plate, these DNA plates are washed in order to measure the pyrophosphate content. This was an extremely important step since it allowed scientists to sequence a wide array of DNA fragments including the publication of the entire human genome project in 2003.
​
After the success of 454, the Solexa method of sequencing became the next major deal. In this method, a flowcell is used, above which complementary oligonucleotides are passed. This method allows for the formation of clonal DNA populations like in the previous method which is both identical to each other. Now, this only creates another DNA strand which can also be called a bridge amplification. The actual sequencing part occurs as fluorescent reversible-terminator dNTP cannot synthesize anymore nucleotides and must take the place of the 3’ hydroxyl group before polymerization occurs. These DNA cells are washed over primed flow cell clusters in cycles and are kept in track by CCD cameras and are passed through fluorophores so that the fluorescent sector of the molecule is extracted in an enzymatic process.
The machines used for genome analyzing allowed scientists to take a look at two ends of the DNA fragments initially but provided a really short reading. The machine used to perform this technique was called the Genome Analyzer. It worked by first allowing a single side of the DNA strand to enter through the machine as it reads the sequences. Afterwards, the second flow cell bound strand is reversed in the opposite direction and read again which complete the paired reading of the DNA strand. Called a paired-end (PE) data, these strands have approximately the same length which allows the genes to line up in close-proximity and provide a ton of information. While reading sequences, this type of a technique eases the process by turning up as reference sequences, repetitive sequences, rearranged DNA or fused genes. This machine was followed by another called a HiSeq which sequenced longer strands of DNA along with more depth. The latter machine, called a MiSeq came at a lower cost with a lower throughput but it made it possible to sequence vast pieces of DNA.
The picture at the top shows the bridge amplification of DNA.
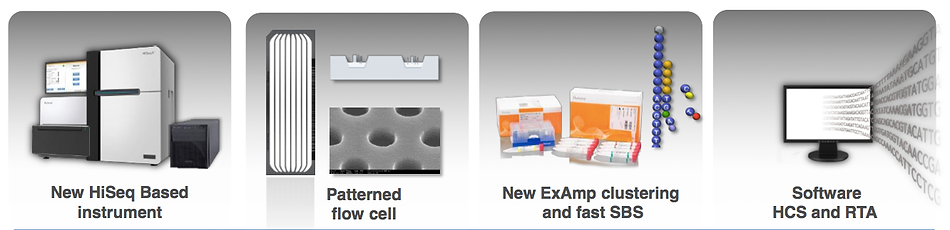
​
Third Generation Sequencing
Third Generation Sequencing is known predominantly for its increased efficacy in the field of genomics and the increased ability to sequence DNA. One of the more recent types is known as a single molecule sequencing (SMS) which does not require any bridge amplification. In this technique, the DNA strand is placed over a planar surface as fluorescent terminator dNTPs go over each base at a time and read it, cleaving it separately each time it does that. This method requires a ton of time since the process does over a base at a time but the fact that it allows for a near-perfect reading of the DNA strands without close to zero errors makes it extremely valuable and expensive as well.
In the third generation sequencing, one of the most predominantly used technology is that of the single molecule real time or just SMRT. In this process, a metallic film with tiny holes covers a chip. As light passes over these holes that are also known as zero-mode waveguides (ZMWs), it causes the particles to radiate at the bottom of the well (this process takes advantage of light properties, which is its longer wavelength in comparison to the smaller diameter that it passes across). Washing DNA over these holes causes them to be trapped in the tiny wells that contain fluorescence. After its done, the positioning of the DNA nucleotides within these wells help indicate their sequences as well.

Citations 1.1 - 1.13
How Relevant is DNA Sequencing?
The completion of the Human Genome Project back in 2003 has made it fairly easy to sequence DNA in the lab. Most labs associated with this field produced over 100,000 billion base sequences on a yearly basis with only a couple of thousand dollars. Most of the support for theses processes come from the National Human Genome Research Institute (NHGRI), the Genome Technology Program and the Advanced DNA Sequencing Technology Awards. One of the goal’s of the NHGRI is to make DNA sequencing as efficient and lower in cost as possible.
​
Watch Richard Resnick, the CEO of GenomeQuest, present a TED Talk on the growing importance of the genomic revolution!
Which New Developments are Being Made?
The next big development in DNA sequencing is leaning towards making a fast enough camera along with a microscope that can see DNA polymerase molecules as they sequence DNA which will give a deeper understanding of what is happening in the machine at that level. Another thought out process is the use of nanopores to sequence DNA. In this process, individual DNA bases are allowed to diffuse or squeeze through a cell membrane as each base is read and interpreted. This interpretation will be made possible by seeing how DNA affects ions and electrical currents flowing through the pore. Further thought applied by companies such as Oxford Nanopore Technologies propose using an artificial pore so that more than one strand can pass through the membrane and can be reused.

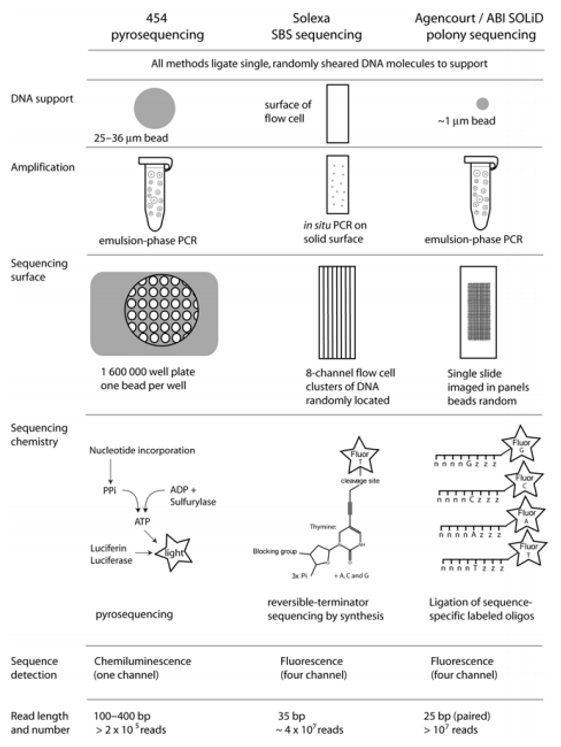
The diagram arrangement at the left shows a few important steps taken during different sequencing methods.
Citations 2.1 - 2.15
Exploring the Depths of Life
​
Sequencing
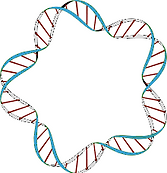